Original article: Methylacidiphilum fumariolicum, astrobiologia ed ambienti estremi, by Luca Tonietti
Astrobiology
Astrobiology, also known as exobiology, aims to study the origin, evolution, distribution, and future of life in the universe. Its fundamental questions have the ultimate goal of defining whether life in space exists, if so, how it works, and finally, how it is detected. Astrobiology uses the most advanced techniques in geology, chemistry, physics, astronomy, and even philosophy. The combined help from these disciplines is necessary to answer the fundamental question: “What is life?”.
The approach “As we know it” is one of the most widespread to searching for life in the universe. What does this mean? Life in the universe – and specifically on the various celestial bodies of our solar system and other systems – could behave as we do on Earth. However, it is essential to underline the term “could” because we can’t be sure that life develops elsewhere in the same way as it happened on the third planet of the solar system – ours.
Why are we talking about such an approach? Because of the carbon chauvinism theorized by Carl Sagan. This thesis predicts that, of all the elements in Mendeleev’s periodic table, only carbon can form a molecular skeleton of every conceivable biochemistry in the universe. According to Sagan, humans, as living organisms based fundamentally on carbon, have not (for the moment) encountered other forms of life outside the planet Earth since it is difficult, if not impossible, to consider different types of biochemistry.
Extreme environments
Let us focus on the solar system. It is well known that in this confined microenvironment (if considered the whole universe), there are conditions that we can define as extreme. Planets like Mercury can be seen as fiery moors, gaseous planets, icy satellites with methane seas, and other compounds that would make it difficult, if not impossible, for human survival. There are places similar to those listed above on Earth, such as deserts, snowy poles, volcanic fumaroles, geysers, etc… Extreme environmental spots where life is being tested. In these natural laboratories, it is possible to study the life that “probably” evolved and developed in space.
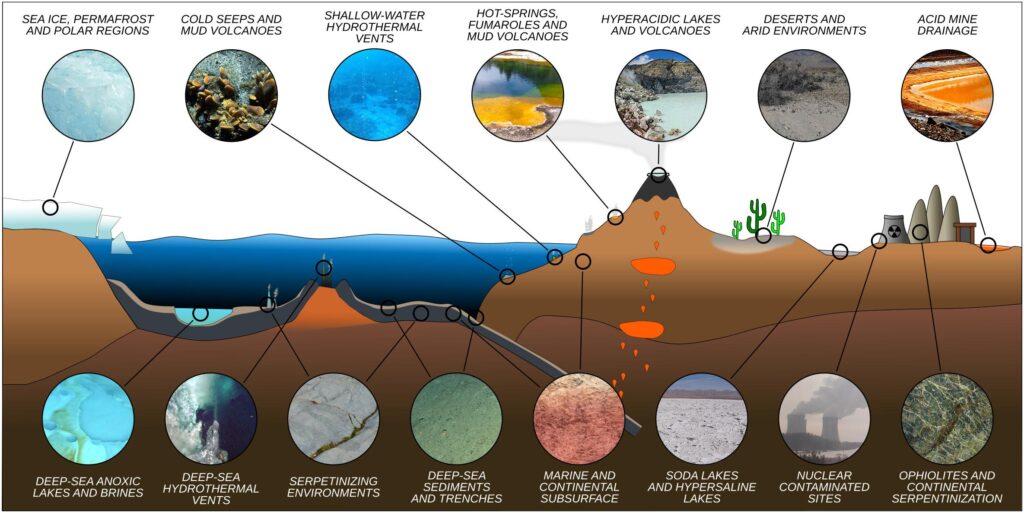
Who inhabits extreme environments? Only tiny microorganisms can survive, which, thanks to their size, can adapt effectively to thrive at low and high temperatures, extreme salinity conditions, highly variable pH, high concentrations of toxic metals, radiation, etc… To give some data:
Biome | Temperature (Celsius) | pH | Pressure (MPa) | Salinity (%NaCl) |
Mine drainage | 1 – 47 | -3.6 – 13.3 | 6 – 14 | 0.008 – 7.6 |
Deserts | -19.4 – 70 | 6.8 – 10 | nr | 0.02 – 30.8 |
Polar regions | -98.6 – 24.3 | 4.6 – 9.6 | 0.1 – 35.5 | 0 – 40.2 |
As shown from the table (Table 1), the ranges are truly extreme compared to an anthropocentric existence. Temperatures starting from below zero, negative pH, high salinity.
The negative pH
Yes, you read well, negative pH. The pH can be negative.
How is it possible to observe a negative pH? In school, we are taught that pH varies on a scale between 0 and 14. However, mathematically speaking, pH can reach negative values being pH = -Log[H+] or pH = ln[H+] * 0.434.
Therefore, in principle, it is possible to calculate negative pH values. However, it is challenging to determine these types of values experimentally. In practice, any acid with a concentration of hydronium ions with molarity greater than 1 could have negative pH values. For example, the pH of a 12M solution of HCl is -1.08.
Methylacidiphilum fumariolicum
Coming back to us, life on Earth can develop in very different environments and under various conditions. Extremophilic organisms adapted over the ages to survive in places that are prohibitive for humans. We will focus on one bacterium in particular, which has managed to internalize elements present in small traces on Earth throughout history.
Before revealing the star; it is known for years that some microorganisms can internalize common metals within their proteins and enzymes. For example, Azotobacter vinelandii (nitrogen fixer), depending on the environment and the relative abundance of metals in the soil, can use as an enzymatic cofactor (of the enzyme nitrogenase), iron, molybdenum, or even vanadium. However, the organism we want to deal with today has done something even more impressive. Methylacidiphilum fumariolicum SoIV can insert inside its particular enzyme ions belonging to lanthanides.
Methylacidiphilum fumariolicum is an autotrophic bacterium first described in 2007 isolated from a mudpot near Naples, Italy. It grows in sulfate mud in a temperature range of 50 to 60 degrees Celsius and a pH between 2 and 5. It can oxidize methane gas, and to fix carbon dioxide, it uses ammonium nitrate or atmospheric nitrogen as its primary source of protons and electrons. Because of the dependence on its methanol dehydrogenase enzyme, Methylacidiphilum fumariolicum can only grow in sites where metals known as rare earth elements (REE) are abundant.
Structural Biochemistry
In general, it has been observed that the methanol dehydrogenase cofactor contains lanthanum. However, it has been shown that it can replace it with other lanthanides, such as cerium, praseodymium, neodymium, samarium, europium, and gadolinium.
Specifically, in 2014, Jahn and coworkers obtained a crystal of the RRE-dependent methanol dehydrogenase (MDH) protein, called XoxF-type. This protein isolated from a Methylacidiphilum fumariolicum SoIV culture in the presence of water and mud from the solfatara near Naples disclosed in it the existence of REE. Cerium proved to be the most abundant element in this experiment.
It is curious noting that the active site structure (Fig. 2) of the REE-dependent MDH is highly conserved compared to the analogous Ca-MDHs, i.e., methanol dehydrogenase dependent on the divalent calcium ion. The only noticeable exception was the presence of an amino acid more (Asp301), which presumably serves to manage the high REE coordination numbers.
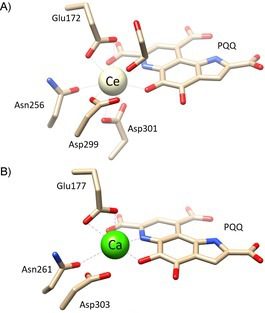
Why are discoveries of this type so unusual and curious?
First of all, the growth parameters of this bacterium already list it among the extreme organisms. Secondly, the presence of REE within the MDH active site is a curious aspect. REE are usually present in minimal traces in terrestrial surface rocks. The fact that they are found within biological systems could be an interesting evolutionary aspect, raising the question: How and why some organisms such as Methylacidiphilum fumariolicum have evolved and adapted to live in “limiting” ecological niches?
Rare Earth Elements characteristics
It is important to emphasize some aspects of REE: like alkali metals and alkaline earth metals, REE are, from a chemical point of view, very electropositive. As a result, they often form ionic bonds in nature and covalent bonds in biological systems. Interestingly, they are also relatively insoluble in water due to their high charge and high ionic potential.
The only exception is the oxidation of uranium in its fully oxidized form +6 – capable of forming soluble ions in the aqueous medium. In the biological world, the MDH is an analog of the one containing bivalent calcium. And even more curious is the fact that this also happens in the abiotic world. Often REE, such as europium and cerium, can replace calcium and aluminum in plagioclase feldspars.
Conclusion
Biochemistry and geochemistry often merged in these organisms on the edge of life, bring to light common points between the biotic and abiotic worlds. Ultimately, it is from inorganic chemistry that organic chemistry came into being.
From a purely astrobiological point of view, “If life on Earth has succeeded in feats unthinkable for humans, who knows how it could have evolved in the cosmos.” “What will we encounter? What will living organisms look like in other solar system objects? What niches will they occupy?” And other questions arise as we discover how, basically, every place on Earth, from the North Pole to the South Pole, has been colonized by some form of life.
Sources
- “About Astrobiology”. NASA Astrobiology Institute and astrobio.it;
- Ward, P. D.; Brownlee, D. (2004). The life and death of planet Earth. New York: Owl Books;
- Joseph Seckbach, Life as We Know It (Cellular Origin, Life in Extreme Habitats and Astrobiology, 10. (2006);
- Sagan, Carl (1973). The Cosmic Connection. Anchor Books (Anchor Press / Doubleday). p. 47;
- Darling, David. “Carbon-based life”. Encyclopedia of Life;
- Pabulo Henrique Rampelotto, Extremophiles and Extreme Environments. Life 2013, 3(3), 482-485;
- (2019). Living at the Extremes: Extremophiles and the Limits of Life in a Planetary Context. Front. Microbiol. 10:780.
- Helmenstine, Anne Marie, Ph.D. “Is Negative pH Possible?” ThoughtCo, Feb. 16, 2021;
- S. E. McGlynn, E. S. Boyd, J. W. Peters, V. J. Orphan. Classifyng the metal dependance of uncharacterized nitrogenases. Front. Microbiol., 30 January 2013;
- Pol, A., et al (2014). “Rare Earth Metals Are Essential for Methanotrophic Life in Volcanic Mudpots”. Environ Microbiol. 16 (1): 255–264;
- Khadem, A. et al (2012). “Genomic and Physiological Analysis of Carbon Storage in the Verrucomicrobial Methanotroph “Ca. Methylacidiphilum Fumariolicum” SolV”. Front Microbiol. 3 (345): 345;
- Khadem, A. et al (2010). “Nitrogen fixation by the verrucomicrobial methanotroph ‘Methylacidiphilum fumariolicum’ SolV”. Microbiology. 156 (1): 1052–9.
- A. M. Ochsner, F. Sontag, M. Buchhaupt, J. Schrader, J. A. Varholt. Appl. Miocrobiol. Biotechnol. 2015, 99, 517-534;
- W. M. White. Geochemistry. Wiley-Blackwell Ed (2017).
Credits figures
- Figure 1 – (N. Merino, H. S. Aronson, D. P. Bojanova, J. Feyhl-Buska, M. L. Wong, S. Zhang, D. Giovannnelli. (2019). Living at the Extremes: Extremophiles and the Limits of Life in a Planetary Context. Front. Microbiol. 10:780).
- Figure 2 – B. Jahn, A. Pol, H. Lumpe, T. R. M. Barends, A. Dietl, C. Hogendoorn, H. J. M. Op den Camp, L. J. Daumann. Similar but Not the Same: First Kinetic and Structural Analyses of a Methanol Dehydrogenase Containing a Europium Ion in the Active Site. ChemBioChem 2018, 19, 1147-1153;
- Featured image – wikipedia.it. https://commons.wikimedia.org/wiki/File:Vulcano.sassi.jpg